- 1College of Life Sciences and Technologies, Tarim University, Alar, China
- 2Laboratory of Systematic & Evolutionary Botany and Biodiversity, College of Life Sciences, Zhejiang University, Hangzhou, China
- 3Department of Botany, University of Wisconsin, Madison, WI, United States
- 4Ministry of Education Key Laboratory for Biodiversity Science and Ecological Engineering, Institute of Biodiversity Science, Fudan University, Shanghai, China
- 5College of Life and Environmental Science, Wenzhou University, Wenzhou, China
Introduction: Phylogenomics have been widely used to resolve ambiguous and controversial evolutionary relationships among plant species and genera, and the identification of unique indels in plastomes may even help to understand the evolution of some plant families. Menispermum L. (Menispermaceae) consists of three species, M. dauricum DC., M. canadense L., and M. mexicanum Rose, which are disjuncly distributed among East Asia, Eastern North America and Mexico. Taxonomists continue to debate whether M. mexicanum is a distinct species, a variety of M. dauricum, or simply a synonym of M. canadense. To date, no molecular systematics studies have included this doubtful species in phylogenetic analyses.
Methods: In this study, we examined phylogenomics and phylogeography of Menispermum across its entire range using 29 whole plastomes of Menispermaceae and 18 ITS1&ITS2 sequences of Menispermeae. We reconstructed interspecific relationships of Menispermum and explored plastome evolution in Menispermaceae, revealing several genomic hotspot regions for the family.
Results and discussion: Phylogenetic and network analyses based on whole plastome and ITS1&ITS2 sequences show that Menispermum clusters into two clades with high support values, Clade A (M. dauricum) and Clade B (M. canadense + M. mexicanum). However, M. mexicanum is nested within M. canadense and, as a result, we support that M. mexicanum is a synonym of M. canadense. We also identified important molecular variations in the plastomes of Menispermaceae. Several indels and consequently premature terminations of genes occur in Menispermaceae. A total of 54 regions were identified as the most highly variable plastome regions, with nucleotide diversity (Pi) values > 0.05, including two coding genes (matK, ycf1), four introns (trnK intron, rpl16 intron, rps16 intron, ndhA intron), and 48 intergenic spacer (IGS) regions. Of these, four informative hotspot regions (trnH-psbA, ndhF-rpl32, trnK-rps16, and trnP-psaJ) should be especially useful for future studies of phylogeny, phylogeography and conservation genetics of Menispermaceae.
Introduction
The moonseed family (Menispermaceae, Ranunculales) includes approximately 72 genera and 526 species. It is most well known as a botanical source for the arrow poison curare (Krukoff and Moldenke, 1938.; Barbosa-Filho et al., 2000; Chen et al., 2013). Many systematic studies have focused on this family, from morphological taxonomic work (Miers, 1851; Prantl, 1888; Diels, 1910; Forman, 1986; Kessler, 1993) to molecular systematics based on plastid sequences (Ortiz et al., 2007; Hoot et al., 2009; Jacques et al., 2011; Wang et al., 2012; Wefferling et al., 2013; Lian et al., 2020), internal transcribed spacer (ITS) (Hong et al., 2001; Wang et al., 2007b), and the combination of morphological and molecular characteristics (Ortiz et al., 2016). The circumscription of tribes/clades within Menispermaceae has been modified several times since the first phylogenetic study using ITS was published (Hong et al., 2001), and ten tribes have been re-delimited in the last few years (Ortiz et al., 2016; Lian et al., 2020). The type genus of the family, Menispermum L., belongs to one of these tribes, Menispermeae DC., together with a second monotypic genus, Sinomenium Diels, represented by S. acutum (Thunb.) Rehder & E.H. Wilson (Ortiz et al., 2016; Lian et al., 2020).
Menispermum, a genus of deciduous climbing woody lianas, is disjuntcly distributed in East Asia, Eastern North America and Mexico (Xiang et al., 2000; Hina et al., 2020; Figure 1). Unlike most members of Menispermaceae, which are endemic to the tropics and subtropics, the ranges of Menispermum extend well into the northern temperate zone. For example, M. dauricum DC. (described in 1817) grows in central to northeastern China, southern Siberia, Korea, and Japan, growing amongst roadside vegetation and/or within open forests (Wu et al., 2008). Menispermum canadense L. (described in 1753) occurs in temperate eastern North America, growing in deciduous woods and thickets (Purrington and Horn, 1993; Morin, 1997). Menispermum mexicanum Rose (published in 1911) is considered to be a third species in the genus because of its larger drupes, glaucous lower leaves and disjunct distribution (northern Mexico) from M. canadense. Although the two species inhabit differ hemispheres, Kundu and Guha (1980) stated that M. mexicanum is most similar to M. dauricum and proposed that M. mexicanum is a variety of M. dauricum, based on detailed morphological and comparative anatomical studies. However, Calderón de Rzedowski (1999) proposed that M. mexicanum is a synonym of M. canadense. Despite its taxonomic uncertainty, M. mexicanum has never been sampled in any published molecular studies.
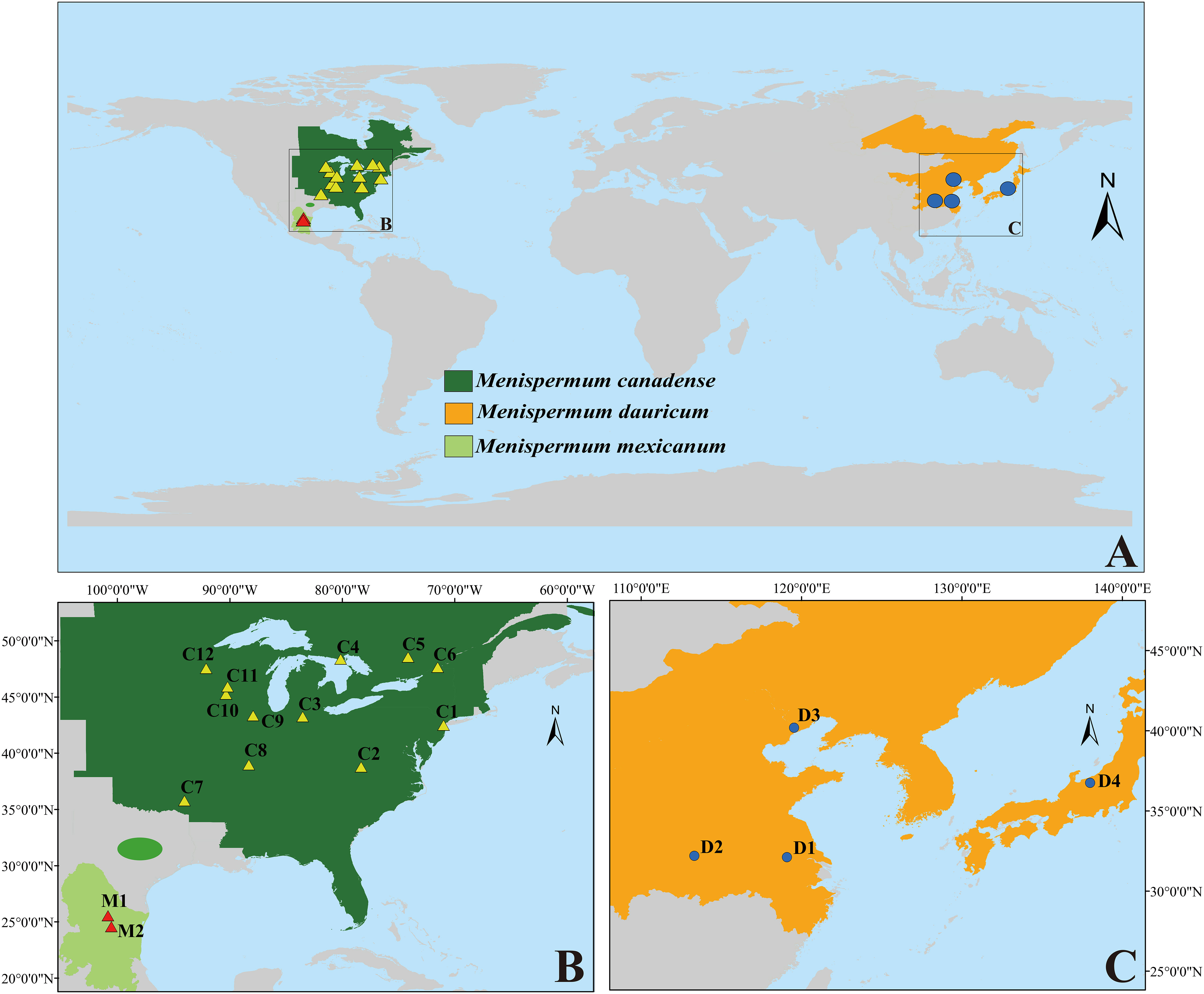
Figure 1 The distribution/sampling sites of M. canadense (dark green/yellow triangle), M. dauricum (orange/blue dot), and M. mexicanum (light green/red triangle). (A) world map; (B) eastern North America; (C) East Asia. Sample codes are the same as in Table 1.
Today there are numerous molecular tools available to plant systematists, and analyses of whole plastomes have become widely used to resolve the circumscription of taxa at different levels of classification within angiosperms, including the families of early-diverging eudicots (Sun et al., 2016), the genera of Ranunculaceae (Liu et al., 2018b; Zhai et al., 2019), and even among species of Aconitum L. (Kong et al., 2017). Comparative plastome analyses also have helped to explore the structural variation of plastomes in angiosperm, such as gene indel (insertion/deletion) events, gene rearrangements, and/or inverted repeat expansion-contraction (Sun et al., 2016; Sun et al., 2018; He et al., 2019; Song et al., 2022). Plastome data can also be a good tool to analyze the phylogeographic patterns among plants (Sun et al., 2018; Demenou et al., 2020; Duan et al., 2020; Xiang et al., 2021). Due to characteristics of plastomes such as maternal inheritance, low to moderate evolutionary rate, their haploid nature which enhances genetic drift, plastome sequences may show a stronger phylogeographical pattern compared to nuclear DNA (Sugiura, 1992; Moore et al., 2010; Demenou et al., 2020; Zhang et al., 2021).
Here, we newly sequenced 19 individuals of Menispermeae, including twelve M. canadense, four M. dauricum, two M. mexicanum and one Sinomenium acutum. Together with ten publicly available plastomes (Arcangelisia gusanlung H.S. Lo, Wen et al., 2021; Fibraurea recisa Pierre, Zheng and Feng, 2022; Pericampylus glaucus (Lam.) Merr., Kang and Wang, 2019; Stephania dielsiana Y.C. Wu; Stephania epigaea H.S. Lo, Guan et al., 2022; Stephania japonica (Thunb.) Miers, Sun et al., 2016; two Stephania tetrandra S. Moore, Cao et al., 2020; Sinomenium acutum, Kim et al., 2020; Tinospora sinensis (Lour.) Merr.), we analyzed a matrix containing a total of 29 plastomes representing seven genera and twelve species. We also analyzed the matrix of ITS1&ITS2 for Menispermum. These results were used specifically to address the following questions: (1) how many species are there in Menispermum? and (2) do significant structural genomic changes appear across the plastomes of Menispermaceae?
Materials and methods
Plant material and DNA extraction
Fresh leaves from three Menispermum species and Sinomenium acutum were collected and then dried in silica-gel. In total twelve samples of M. canadense, four of M. dauricum, and one Sinomenium acutum were included. Voucher specimens were mostly deposited at the Herbarium of Zhejiang University (HZU), with the two M. mexicanum specimens (one of which is an isotype specimen) were sampled from the Gray Herbarium (GH) and Arnold Arboretum Herbarium (A) of Harvard University (Table 1). Total genomic DNA was extracted using DNA Plantzol Reagent (Invitrogen, Carlsbad, CA, United States) according to the manufacturer’s protocol. Agarose gel electrophoresis and an ultraviolet spectrophotometer (K5800, KAIAO, Beijing, China) were used to check the quality and quantity of genomic DNA, respectively.
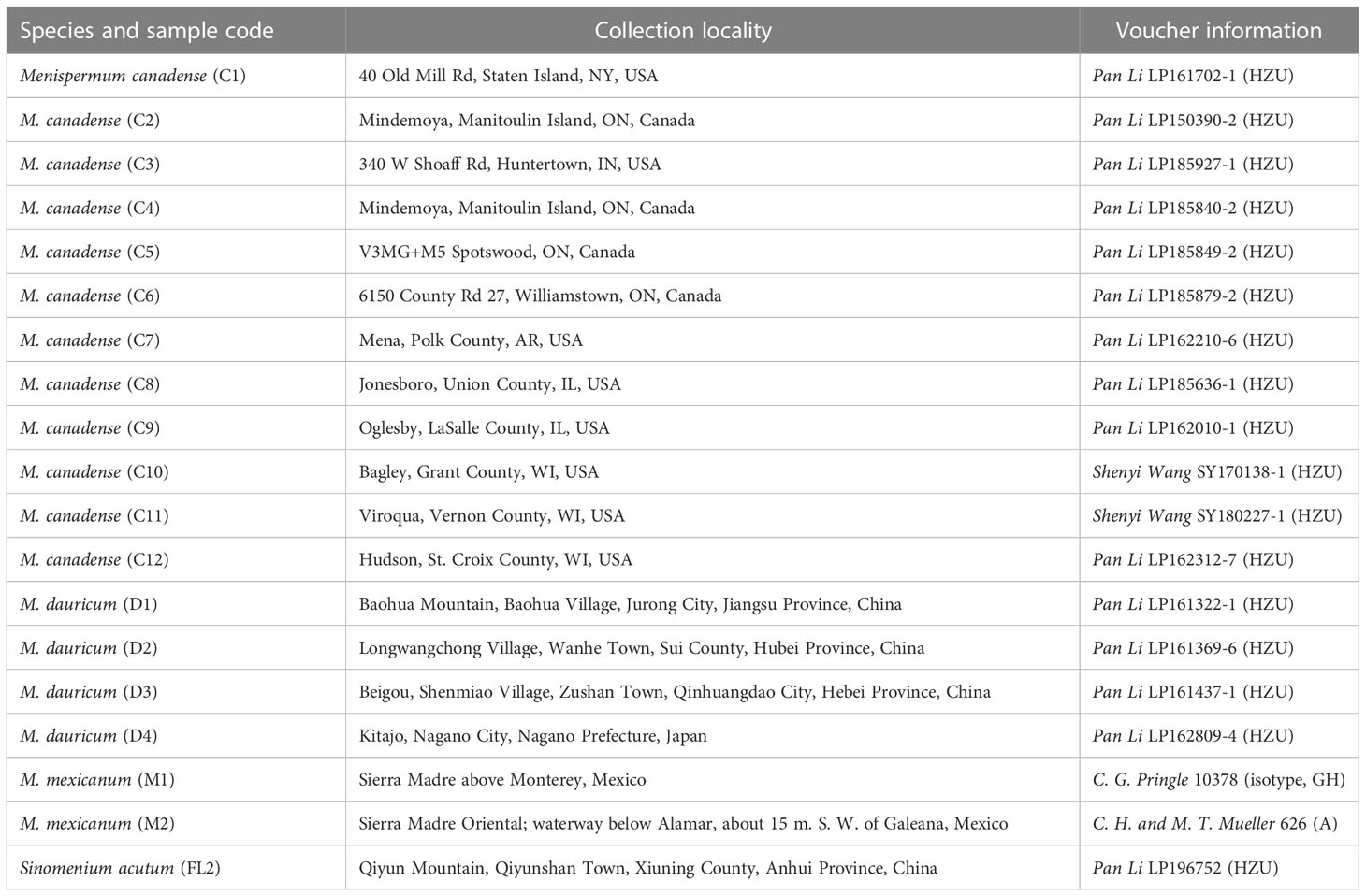
Table 1 Collection locality and voucher information of Menispermeae samples newly sequenced in this study.
Genome sequencing, assembly, and annotation
Short-inserts of 500-bp paired-ends were used to construct the libraries by Genomic DNA Sample Prep Kit (Illumina, San Diego, CA, United States). We used tags to index DNA from each species and pooled samples together for sequencing on a HiSeqTM 2500 platform at the Beijing Genomics Institute (BGI, Shenzhen, China) to obtain clean reads of each sample. Then, these reads were assembled into contigs or plastid sequences using GetOrganelle v 1.7.5.3 (Jin et al., 2020), and visualized in Bandage v 0.8.1 (Wick et al., 2015) to identify whether these contigs or plastid sequences were the whole plastome for each sample. Additionally, due to low sequencing depth of two M. mexicanum herbarium samples, only small size of contigs were obtained by GetOrganelle. To obtain longer plastid sequences, these short contigs were mapped to the reference M. dauricum (MH298220, Hina et al., 2018). Yet, there are still a lot of gaps. To obtain these gap-sequences, NOVOPlasty v 4.2 (Dierckxsens et al., 2017) was applied to assemble these sequences,with the neighboring protein-coding or tRNA genes in the plastome reference corresponding these gap sequences as seeds, and using the same plastome reference above. At last, these newly assembled gap-sequences were inserted into the above longer plastid sequences of M. mexicanum using software Geneious Prime® 2021.2.2 (www.geneious.com) and we eventually generated their whole plastomes. Plastomes were annotated using ‘2544-plastome’ dataset of CPGAVAS2 (Shi et al., 2019), with default setting. Then, these whole plastomes were illustrated with the online tool OrganellarGenome DRAW v1.3.1 (Greiner et al., 2019) and deposited in GenBank (Table 1). We also downloaded ten additional whole plastomes of Menispermaceae from NCBI, i.e., A. gusanlung (MW829779), F. recisa (OK539642); T. sinensis (MN727386), P. glaucus (MN539265), S. dielsiana (MW1453970), S. epigaea (MZ678241), S. japonica (KU204903), S. tetrandra (MT849286, MT859132), Sinomenium acutum (MN626719) and reannotated these plastomes to perform a comparative plastome analysis of these Menispermaceae. The 18 ITS1&ITS2 were also assembled using GetOrganelle, but M. mexicanum (M2) were failed.
Comparative plastome analyses
Altogether, by combining new and previously published plastomes (Table 1), a total of 12 plastomes representing 12 species of Menispermaceae were used to investigate the plastome evolution in this family. Interspecific variation was documented using mVISTA (Frazer et al., 2004) in Shuffle-LAGAN mode. Rearrangements of plastomes were checked by Mauve 2.3.0 (Darling et al., 2004). Gene and structure differences in the junctions of single copies and inverted repeat regions were visualized and compared through the software IRscope (Amiryousefi et al., 2018). The protein-coding sequences (CDS), intergenic spacer (IGS) and intron regions were extracted sequentially according to the criterion that alignment length > 200 bp and containing at least one mutation to estimate the nucleotide diversity (Pi) of plastid sequences of Menispermaceae. Pi value of each sequence was calculated in DNASP v 6.12.03 (Rozas et al., 2017).
Phylogenetic and phylogeographic analyses
The phylogeny of Menispermaceae based on whole plastome/nrDNA was inferred using maximum likelihood (ML) and Bayesian inference (BI) methods via RAxML-HPC2 on XSEDE v 8.2.12 (Stamatakis, 2014) on CIPRES Science Gateway website (https://www.phylo.org) and MrBayes v 3.2.6 (Ronquist et al., 2012), respectively. For ML analysis, we set 1000 bootstrap replicates but used defaults for the other parameters. For BI analysis, we first evaluated the proper model of evolution (GTR+F+I+G4 for ptDNA, GTR+F+I for nrDNA) based on the Akaike Information Criterion (AIC) in ModelFinder (Kalyaanamoorthy et al., 2017), then ran two independent Markov chain Monte Carlo (MCMC) chains, with a set of 1,000,000 generations for each chain, and sampled one time for every 1,000 generations; the first 25% of the trees were discarded.
For phylogeographic analyses, we focused only on the 18 samples of Menispermum. The 18 plastomes were aligned with defaulted parameters by the plug-in of MAFFT in Geneious Prime® 2021.2.2, and inferred the number of haplotypes (Nh) (excluding sites with gaps/missing data) in DNASP. The genealogical relationships of haplotypes were identified via TCS haplotype network using the software PopART v 1.7 (Leigh et al., 2015). The 17 ITS1&ITS2 sequences of Menispermum were analyzed using the same methods.
Results
Plastome features
Total coverage of the 19 newly sequenced plastomes ranged from 9 × [M. mexicanum (M2)] to 536.9 × [M. canadense (C10)] (Table 2). These whole plastomes of Menispermaceae show a typical angiosperm quadripartite structure, including a pair of IR regions (IRa and IRb) and two single copy regions (LSC and SSC) (Figure 2). All the plastomes contain 114 unique genes, consisting of 80 CDS genes, 30 tRNA genes, and four rRNA genes (Table S1). The sizes of these newly assembled whole plastomes of Menispermeae range from 160,185 bp [M. dauricum (D4)] to 163,171 bp [M. mexicanum (M2)], and LSC, SSC and IR ranged from 89,380 bp in M. dauricum (D4) to 91,765 bp in M. mexicanum (M2), 20,781 bp in M. dauricum (D1) to 21,286 bp in M. canadense (C1), and 24,887 bp in M. dauricum (D1) to 25,064 bp in M. dauricum (D3), respectively (Table 2). The GC content of the 19 plastomes ranges from 37.7% to 38.4%, with the values in the IR regions (43.4–43.7%) being the greatest, followed by the LSC (35.8–36.6%) and SSC (32.1–33.4%).
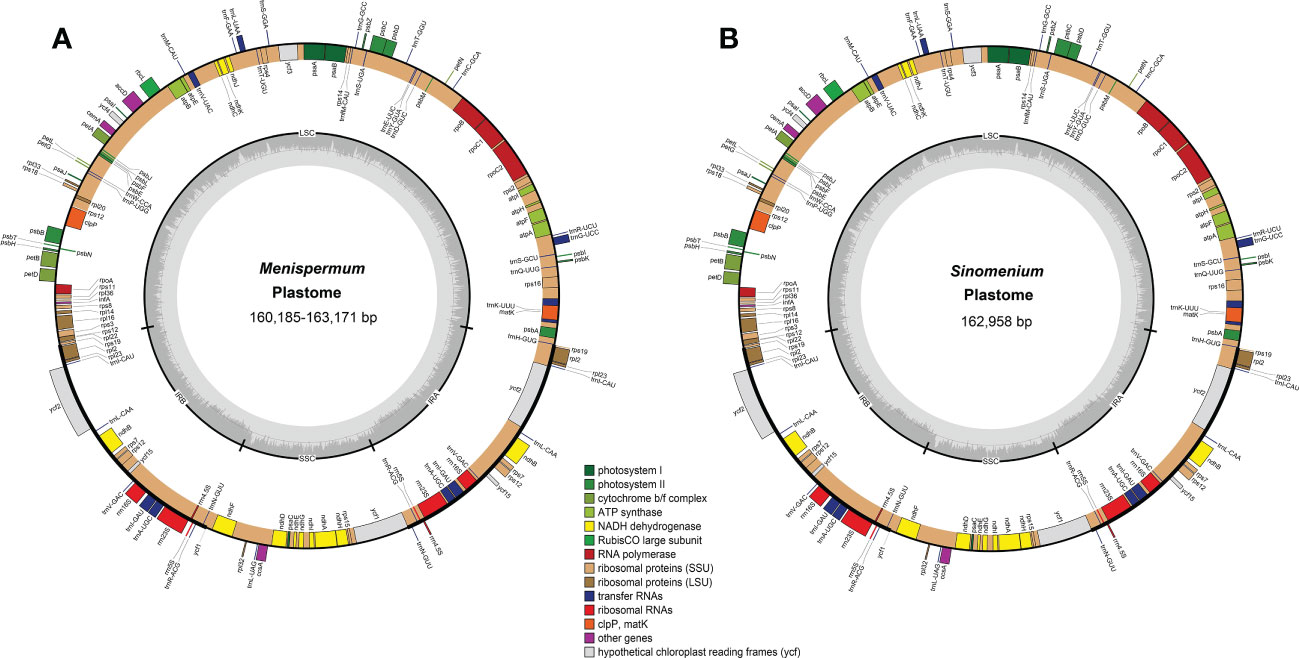
Figure 2 Representative plastome maps of the newly sequenced plastomes of Menispermaceae (19 in total). (A) Menispermum; (B) Sinomenium.
Phylogenetic and the phylogeographic analyses
The phylogenetic trees we generated using ML and BI methods resulted in similar topologies with high support (Figure 3). The Coscinieae + Burasaieae clade is the first diverging lineage of the family. This is followed by the clade of Anomospermeae + Cissampelideae as sister to tribe Menispermeae, within which Sinomenium is sister to the species of Menispermum. Menispermum dauricum (Clade A) is monophyletic and sister to the Clade B of M. canadense + M. mexicanum. Clade B is divided into two highly supported clades, Clade B1 (BS = 90, PP = 1) and Clade B2 (BS = 90, PP = 1) each of which contains accessions of both M. mexicanum and M. canadense, rendering them not monophyletic. The ML and BI trees based on the ITS1&ITS2 sequences show similar topology with the plastid tree, i.e., Menispermum dauricum (clade a) is sister to clade b that consists of M. canadense and M. mexicanum (Figure 4). In clade b, M. mexicanum (M1) was nested in clade b1 which is sister to clade b2.
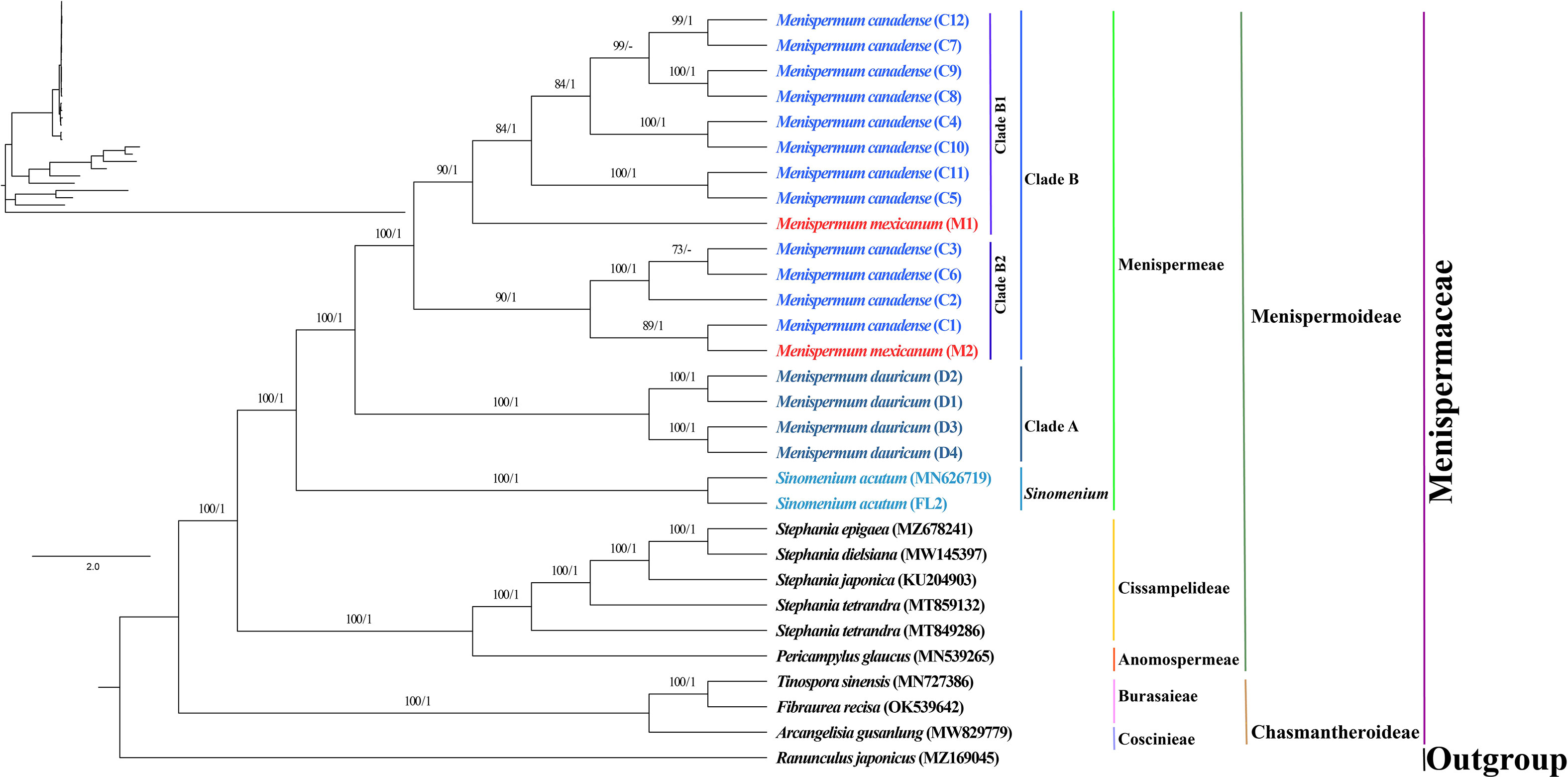
Figure 3 Maximum likelihood (ML) and Bayesian inference (BI) phylogeny based on 29 complete plastomes of Menispermaceae, with Ranunculus as outgroup. Numbers at each node represent ML bootstrap support (BS) and BI posterior probability (PP) values, respectively. Hyphens indicate the nodes not found in the strict consensus BI tree. The phylogram on the upper left shows the relative branch lengths. Sample codes are the same as in Table 1.
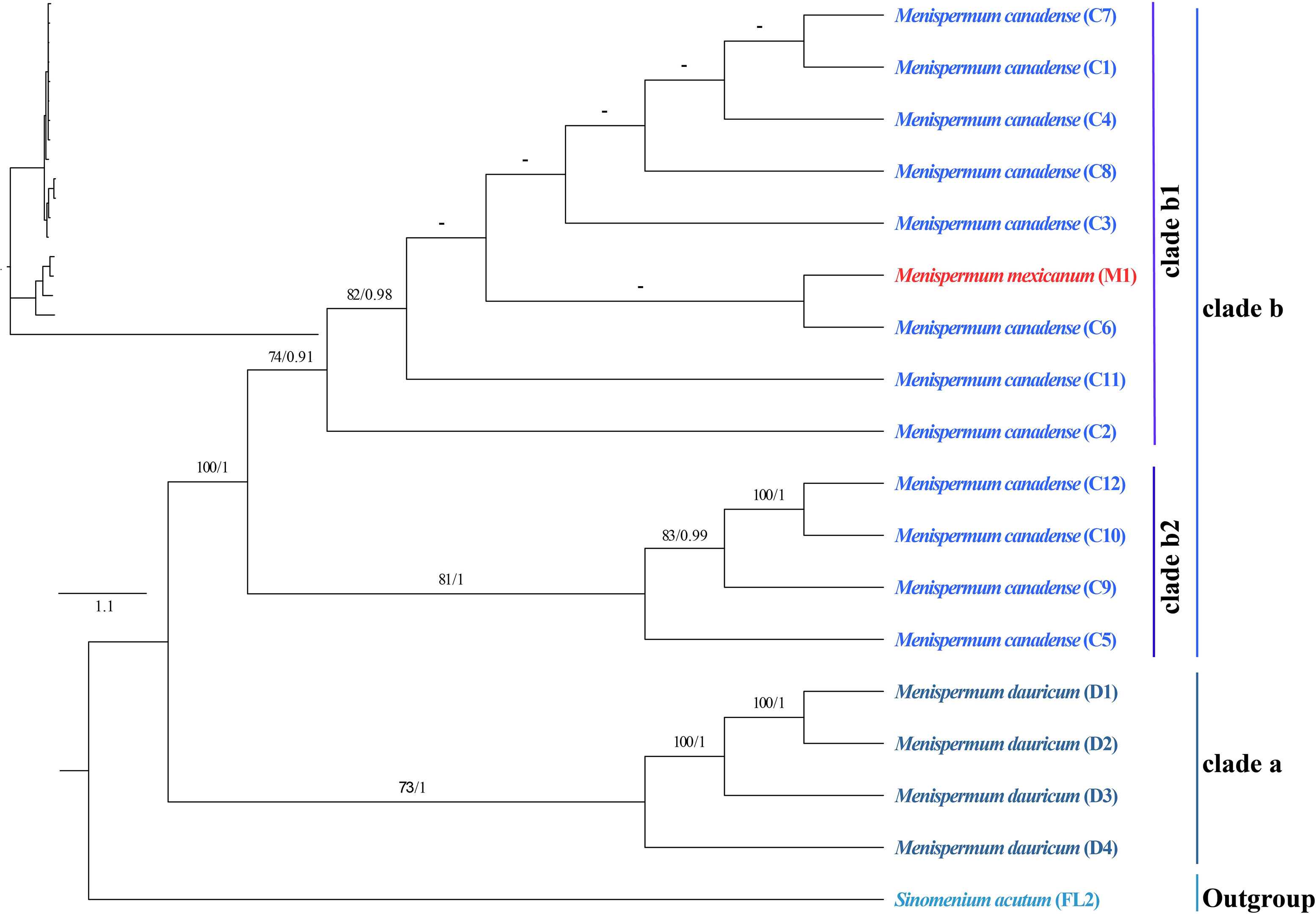
Figure 4 Maximum likelihood (ML) and Bayesian inference (BI) phylogeny based on 17 ITS1&ITS2 sequences of Menispermum, with Sinomenium as outgroup. Numbers at each node represent ML bootstrap support (BS) and BI posterior probability (PP) values, respectively. Hyphens indicate the nodes not found in the strict consensus BI tree. The phylogram on the upper left shows the relative branch lengths. Sample codes are the same as in Table 1.
The 18 whole plastomes of Menispermum were identified to have 15 haplotypes (Figure 5A). All of these are unique except that haplotypes H3, H7 and H10 are shared in two samples (Figure 5A). The 17 ITS1&ITS2 sequences of Menispermum were identified to have 15 ribotypes (Figure 5B). All of these are unique except that ribotype R5 is shared by three samples (C3, C4, C8) (Figure 5B). The haplotypes and ribotypes cluster significantly into two lineages, i.e. M. dauricum and M. canadense + M. mexicanum. Yet, the haplotypes and ribotype of M. mexicanum sampled are all embedded within M. canadense.
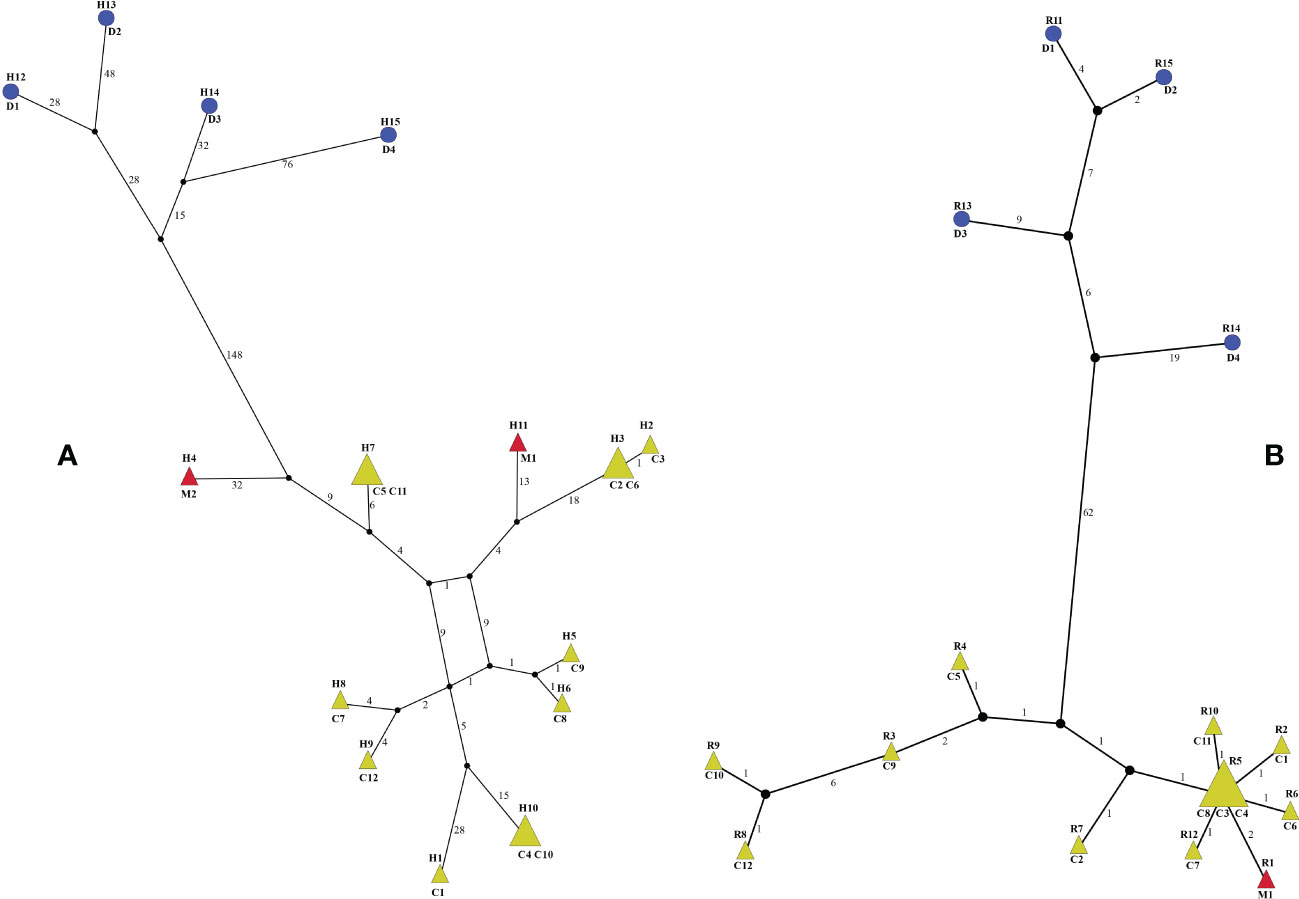
Figure 5 Haplotype (A) and ribotype (B) networks of Menispermum. C, D, and M represent M. canadense, M. dauricum, and M. mexicanum, respectively. Sample codes are the same as in Table 1.
Comparative plastome analyses
Due to minor variations in each species, we performed a comparative plastome analysis of Menispermaceae using only 12 plastomes representing 12 species. The Stephania species have the shortest plastomes (~157 kb), whereas species of Menispermeae have the longest (~163 kb). Global visualization using mVISTA and MAUVE showed that all of these 12 plastomes of Menispermaceae have a consistent gene order, except that the rpoC2 gene in the plastome of T. sinensis is inverted (Figures S1, S2). The rps19 gene of F. recisa is located in LSC, with a 32 bp-distance away from the junction of LSC and IRb (JLB), whereas the other eleven species have their rps19 located at the boundary of LSC/IRb (JLB) and the genes copied 71–174 bp sequences (ψrps19) in IRa (Figure 6). The junction of SSC/IRa (JSA) in the all 12 plastomes are located in ycf1 gene (5574 bp- 5615bp), and the gene copied different length of sequences (ψycf1) in IRb, which ranged from 18 bp in F. recisa to 483 bp in S. dielsiana (Figure 6). Especially, only two species (S. dielsiana and S. epigaea) have their ndhF gene across the SSC/IRB boundary (JSB).
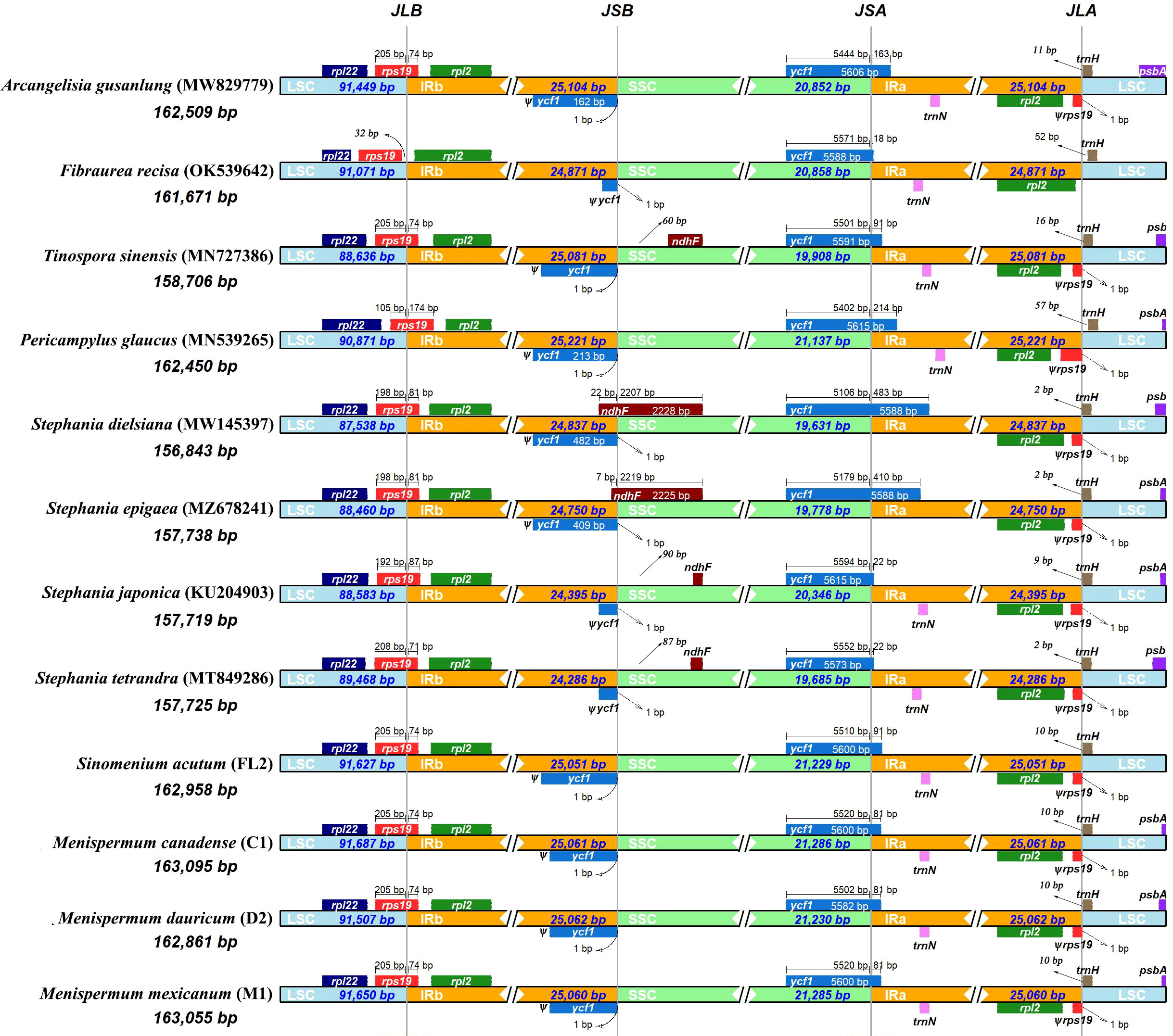
Figure 6 Comparison of the LSC/IRb/SSC/IRa junctions among the 12 complete plastomes of Menispermaceae. Sample codes are the same as in Table 1.
We also calculated the length of all genes across 29 plastomes in Menispermaceae. Of all 114 genes, 26 genes varied in length, and only minor variations (less than 42 bp) were documented among 18 genes, i.e. matK, atpF, rpoC1, rpoB, ndhK, atpE, accD, rps18, rpl20, petD, rpoA, infA, rpl23, ndhF, rpl32, ccsA, rps15, ycf1 (Table S2). The rpoC2 gene in the plastome of T. sinensis occurred a base replace from “T” to “C” in the locus of 2137 bp, which results in a premature termination of that gene, about 2,000 bp shorter than the other plastomes. The ycf2 gene in all Stephania plastomes has an approximately 417 bp deletion at locus 1787/1788 bp, compared to the plastomes from the other genera. In the plastome of P. glaucus, the ycf15 gene is about 200 bp shorter than the other 28 Menispermaceae plastomes because of a 10-bp insertion of “TATTCTATTA” in the locus of 211 bp, resulting in a premature termination. The sequences in the second extron of rps16 gene in S. dielsiana and S. epigaea, are significantly different from the other 27 plastomes, with “AGAATAAAA” and “AGAATAAAT” replacing “GT” or “AT” in the loci of 103-111 bp, which results in the rps16 gene being almost halved in the two plastomes, compared to the remaining plastomes.
Two single-copy (SSC, LSC) regions exhibit a higher level of sequence variation than the IR regions (Figure 2). In the alignment of the 12 plastomes of Menispermaceae, a total of 147 regions were extracted to calculate the value of nucleotide diversity (Pi values). They included 65 intergenic spacer (IGS) regions, 63 protein-coding (CDS) regions, 17 intron regions (of CDS/tRNA genes), and two rRNA gene (Figure 7). A total of 54 regions with Pi more than 0.05 in LSC or SSC were revealed (Figure 7). The Pi in CDSs and intron regions showed significantly lower than those in IGS regions. These CDSs ranged from 0.00317 (rps7) to 0.06347 (ycf1), only matK and ycf1 genes showed high values more than 0.05. For the intron regions, Pi ranged from 0.00436 (trnA intron) to 0.06359 (ndhA intron), four introns (trnK, rpl16, rps16 and ndhA) gene showed high diversity (Pi > 0.05). Besides, for the 65 IGS regions, the value of Pi ranged from 0.00631 (ndhB-rps7) to 0.14317 (trnS-trnG), 48 regions showed high diversity, and six showed remarkably high diversity (Pi > 0.1; i.e., trnS-trnG, trnH-psbA, ndhF-rpl32, trnK-rps16, ccsA-ndhD, trnP-psaJ; see Figure 5). The sizes and Pi values of the six hotspot regions are shown in Table S3, and corresponding phylogenetic trees based on each region are shown in Figure S3.
Discussion
Phylogenetic, phylogeographic and taxonomic inferences
Our molecular phylogeny of Menispermaceae (represented by five tribes, seven genera, twelve species and 29 accessions) fully resolves the relationships among these taxa with robust support and bifurcates into two major clades that correspond to the subfamilies Chasmantheroideae and Menispermoideae (Figure 3). Within the latter subfamily, the tribe Menispermeae is sister to the (Cissampelideae + Anomospermeae) clade, consistent with previous studies based on plastid sequences (rbcL, atpB, matK, ndhF and trnL-F) and/or combined plastid+nuclear ITS region (Ortiz et al., 2007; Ortiz et al., 2016; Wang et al., 2007b; Wang et al., 2012; Wefferling et al., 2013; Ortiz et al., 2016) (Figure 3).
Within tribe Menispermeae, Sinomenium is sister to Menispermum with maximum support (BS = 100, PP = 1, Figure 3), consistent to the previous results based on plastid and/or combined rDNA sequences (Hoot et al., 2009; Jacques et al., 2011; Wang et al., 2012; Wefferling et al., 2013; Ortiz et al., 2016; Lian et al., 2020). The species M. dauricum (Clade A) from East Asia is monophyletic, and the two accessions from eastern (D1) and central China (D2) clustered into a subclade, whereas the remaining two from northern China (D3) and Japan (D4) clustered into another subclade (Figure 1 and Table 1). A similar lineage divergence between north and south has been found in unrelated plants such as Saxifraga sect. Irregulares (Zhang et al., 2019) indicating that this pattern is not random. Clade B (Figure 3) contains the accessions of M. canadense from the eastern United States and Canada plus two accessions of M. mexicanum from northern Mexico, which are not sister to each other. Based on the holotype specimen (U. S. National Herbarium no. 462662) collected by Dr. C. G. Pringle in the Sierra Madre, Mexico on July 9th, 1907, Rose (1911) proposed a new species, M. mexicanum, because of its leaves not being glaucous beneath, larger drupes, and a much more southern range than M. canadense. However, Kundu and Guha (1980) considered M. mexicanum to be a variety of Asian M. dauricum based on their detailed morphological and comparative anatomical studies. Our study is the first molecular systematics study that includes all three species. Both accessions of M. mexicanum are from different herbarium specimens collected in Mexico (they cannot be misidentifications of M. canadense) and one of them M. mexicanum (M1) was sampled directly from a historical isotype specimen. These two accessions of M. mexicanum are nested within M. canadense in both phylogenetic tree (Figures 3, 4) and TCS diagram (Figure 5), rendering neither species monophyletic. Hence, we here propose that M. mexicanum is merely a geographic disjunct population and a synonym of M. canadense. This result supports the taxonomic treatment published by Calderón de Rzedowski (1999).
Comparative plastome analyses
All of the 19 newly sequenced plastomes of Menispermeae in our study contain 114 unique genes (Table S1 and Figures S1, S2), consistent with the eleven previously reported Menispermaceae plastomes (Sun et al., 2016; Hina et al., 2018; Kang and Wang, 2019; Cao et al., 2020; Kim et al., 2020; Wen et al., 2021; Guan et al., 2022; Zheng and Feng, 2022). However, these plastomes have two more genes (ycf15, rpl32) than the tribe Anemoneae, and one more gene (ycf15) than the genera Anemoclema (Franch.) W.T. Wang (Jiang et al., 2017), Archiclematis Tamura, Clematis L., and Naravelia DC. of Ranunculaceae, the sister family of Menispermaceae (Kim et al., 2004; Liu et al., 2018a).
The rpoC2 gene in T. sinensis contains an inversion and significant truncation of about 2 kb (Table S2 and Figure S2), which is possibly due to the base substitution of “T/C” in the locus 2,137 bp and resulting in the premature termination of that gene. The pseudogene ψycf1 of S. dielsiana and S. epigaea, which may have resulted from an IR expansion, is ~200 bp longer than that of the ten other Menispermaceae species (Song et al., 2022). The rps19 gene of F. recisa is located in the LSC region, resulting in the fact that ψrps19 does not appear in IRa. This phenomenon also has been observed in other plant families, such as some species of Aconitum (Ranunculaceae), Dicorynia paraensis Benth. (Fabaceae), and at least three species of Oxalis L. (Oxalidaceae) (Kong et al., 2017; Bai et al., 2021; Li et al., 2021).
The plastome length of our twelve focus species shows significant differences (Figure 6), ranging from 156,843 bp (Stephania dielsiana) to 163,095 bp [M. canadense (C1)], which is mainly ascribed to insertions/deletions (indels) in the intergenic spacer regions. Yet, some gene length differences are significant between tribes or genera, and even among species. For example, an ~417 bp deletion within the ycf2 gene is present in all Stephania plastomes. A 12 bp deletion in the atpF gene, which may be a molecular synapomorphy of the tribe Menispermeae, occurs only in the genera Sinomenium and Menispermum. For Menispermum, the ndhF gene in four M. dauricum plastomes has a 6 bp insertion compared with those from M. canadense and M. mexicanum. Indels and consequently premature termination of various genes were also found in the plastome evolution of Menispermeae, such as an about 200 bp truncation of P. glaucus caused by the 10-bp insertion of “TATTCTATTA”. In brief, indels and premature termination events of genes are usual phenomenon of plastome evolution in angiosperms and we have documented them within Menispermaceae as well (Wang et al., 2007a; Fu et al., 2017; Wang et al., 2020; Song et al., 2022).
Highly variable regions of Menispermaceae plastomes
Several plastid gene and spacer sequences (atpB, matK, ndhF, rbcL, and trnL-F) have been used to resolve the backbone phylogeny of Menispermaceae (Ortiz et al., 2007; Jacques et al., 2008; Hoot et al., 2009; Jacques et al., 2011; Wang et al., 2012; Wefferling et al., 2013; Ortiz et al., 2016; Lian et al., 2020). However, only 63 of 72 genera and fewer than 150 of 526 species were sampled in those previous studies (Hong et al., 2001; Ortiz et al., 2007; Wang et al., 2007b; Hoot et al., 2009; Jacques et al., 2011; Wang et al., 2012; Wefferling et al., 2013; Lian et al., 2020), suggesting that additional sampling of both taxa and data might be needed to get a full picture of evolutionary relationships within the family. In this study, of the six newly proposed hotspot regions, with nucleotide diversity (Pi) values > 0.1 just four loci (trnH-psbA, ndhF-rpl32, trnK-rps16 & trnP-psaJ) are adequate to recover the monophyly of all genera and species that are represented by more than one individual (Table S3). This is encouraging and suggest that these markers will be useful candidate DNA barcodes for further studies on phylogeny and phylogeography of Menispermaceae (Barbosa-Filho et al., 2000; Singh and Bedi, 2016; He et al., 2018; Hina et al., 2020; Hao et al., 2022).
Data availability statement
The data presented in the study are deposited in the NCBI repository, accession number OP271866-OP271873, OP271875-OP271884, OP980572, OQ198585-OQ198602, OQ198604-OQ198621..
Author contributions
PL and ZY designed this study. PL and SW collected plant materials. SS and ZY assembled and analyzed the data, and prepared the figures and tables. ZY, SS, and PL wrote the original draft, KC, YW, and XJ modified the manuscript. All authors contributed to the article and approved the submitted version.
Funding
This research was supported by the National Natural Science Foundation of China (Grant Nos. 31970225 & 32060053), and the NSFC-NSF Dimensions of Biodiversity Program (Grant No. 31461123001).
Acknowledgments
We sincerely thank Harvard University Herbaria for allowing sampling from herbarium specimens, and Dr. Ruisen Lu (Nanjing Botanical Garden Memorial Sun Yat-Sen), Dr. Chih-Chieh Yu (Xishuangbanna Tropical Botanical Garden) and Dr. Zhechen Qi (Zhejiang Sci-Tech University) for their help with sample collection in the wild.
Conflict of interest
The authors declare that the research was conducted in the absence of any commercial or financial relationships that could be construed as a potential conflict of interest.
Publisher’s note
All claims expressed in this article are solely those of the authors and do not necessarily represent those of their affiliated organizations, or those of the publisher, the editors and the reviewers. Any product that may be evaluated in this article, or claim that may be made by its manufacturer, is not guaranteed or endorsed by the publisher.
Supplementary material
The Supplementary Material for this article can be found online at: https://www.frontiersin.org/articles/10.3389/fpls.2023.1116300/full#supplementary-material
Supplementary Figure 1 | Comparison of the 12 plastomes of Menispermaceae analyzed in this study using mVISTA, with Ranunculus japonicus (MZ169045) as a reference.
Supplementary Figure 2 | MAUVE alignment of the 12 Menispermaceae plastomes analyzed in this study, with Arcangelisia gusanlung as a reference.
Supplementary Figure 3 | Maximum likelihood (ML) trees based on each of the 6 hotspot regions (Pi > 0.1). (A) trnS-trnG; (B) trnH-psbA; (C) ndhF-rpl32; (D) trnK-rps16; (E) ccsA-ndhD; (F) trnP-psaJ. Numbers listed at each node represents bootstrap support (BS) values. The hyphen indicates BS < 50%.
References
Amiryousefi, A., Hyvonen, J., Poczai, P. (2018). IRscope: an online program to visualize the junction sites of chloroplast genomes. Bioinformatics 34, 3030–3031. doi: 10.1093/bioinformatics/bty220
Bai, H. R., Oyebanji, O., Zhang, R., Yi, T. S. (2021). Plastid phylogenomic insights into the evolution of subfamily Dialioideae (Leguminosae). Plant Diversity 43, 27–34. doi: 10.1016/j.pld.2020.06.008
Barbosa-Filho, J. M., Da-Cunha, E. V. L., Gray, A. I. (2000). Alkaloids of the Menispermaceae (San Diego: Academic Press).
Calderón de Rzedowski, G. (1999). “Familia Menispermaceae,” in Flora del bajío y regiones adyacentes, vol. 72 . Ed. Rzedowski, J., Calderón de Rzedowski, G. (Paátzcuaro: Instituto de Ecología A.C.), 1–13.
Cao, L., Mu, Z., Sheng, S., Chen, Y., Zhong, G., Du, X. (2020). The complete chloroplast genome of Stephania tetrandra (Menispermaceae). Mitochondrial DNA B Resour. 5, 3819–3820. doi: 10.1080/23802359.2020.1840935
Chen, Q., Zhang, J., Zhang, W., Chen, Z. (2013). Analysis of active alkaloids in the Menispermaceae family by nonaqueous capillary electrophoresis-ion trap mass spectrometry. J. Separation Sci. 36, 341–349. doi: 10.1002/jssc.201200678
Darling, A. C., Mau, B., Blattner, F. R., Perna, N. T. (2004). Mauve: multiple alignment of conserved genomic sequence with rearrangements. Genome Res. 14, 1394–1403. doi: 10.1101/gr.2289704
Demenou, B. B., Migliore, J., Heuertz, M., Monthe, F. K., Ojeda, D. I., Wieringa, J. J., et al. (2020). Plastome phylogeography in two African rain forest legume trees reveals that dahomey gap populations originate from the Cameroon volcanic line. Mol. Phylogenet. Evol. 150, 106854. doi: 10.1016/j.ympev.2020.106854
Diels, L. (1910). “Menispermaceae,” in Das pflanzenreich IV, vol. 94 . Ed. Engler, A. (Leipzig: Wilhelm Engelmann press).
Dierckxsens, N., Mardulyn, P., Smits, G. (2017). NOVOPlasty: de novo assembly of organelle genomes from whole genome data. Nucleic Acids Res. 45, e18. doi: 10.1093/nar/gkw955
Duan, L., Harris, A. J., Su, C., Zhang, Z. R., Arslan, E., Ertugrul, K., et al. (2020). Chloroplast phylogenomics reveals the intercontinental biogeographic history of the liquorice genus (Leguminosae: Glycyrrhiza). Front. Plant Sci. 11. doi: 10.3389/fpls.2020.00793
Forman, L. L. (1986). “Menispermaceae,” in Flora malesiana, vol. 10 . Eds. van Steenis, C. G. G. J., de Wilde, W. J. J. O. (Netherlands, Dordrecht: Kluwer Press), 157–253.
Frazer, K. A., Pachter, L., Poliakov, A., Rubin, E. M., Dubchak, I. (2004). VISTA: computational tools for comparative genomics. Nucleic Acids Res. 32, W273–W279. doi: 10.1093/nar/gkh458
Fu, C. N., Li, H. T., Milne, R., Zhang, T., Ma, P. F., Yang, J., et al. (2017). Comparative analyses of plastid genomes from fourteen Cornales species: inferences for phylogenetic relationships and genome evolution. BMC Genomics 18, 956. doi: 10.1186/s12864-017-4319-9
Greiner, S., Lehwark, P., Bock, R. (2019). OrganellarGenomeDRAW (OGDRAW) version 1.3.1: expanded toolkit for the graphical visualization of organellar genomes. Nucleic Acids Res. 47, W59–W64. doi: 10.1093/nar/gkz238
Guan, Q., Feng, D., Fan, M. (2022). The complete chloroplast genome sequence of the medicinal plant Stephania epigaea H. S. Lo 1978 (Menispermaceae) from yunnan, China. Mitochondrial DNA B Resour. 7, 1403–1405. doi: 10.1080/23802359.2022.2104670
Hao, D. C., Xu, L. J., Zheng, Y. W., Lyu, H. Y., Xiao, P. G. (2022). Mining therapeutic efficacy from treasure chest of biodiversity and chemodiversity: Pharmacophylogeny of Ranunculales medicinal plants. Chin. J. Integr. Med 28, 1111–1126. doi: 10.1007/s11655-022-3576-x
He, Y., Guo, C. X., Zeng, X. Y., Yang, H., Xiong, X. Y., Qiu, P. (2018). Genetic population variation and phylogeny of Sinomenium acutum (Menispermaceae) in subtropical China through chloroplast marker. BioRxiv. doi: 10.1101/449900
He, J., Yao, M., Lyu, R. D., Lin, L. L., Liu, H. J., Pei, L. Y., et al. (2019). Structural variation of the complete chloroplast genome and plastid phylogenomics of the genus Asteropyrum (Ranunculaceae). Sci. Rep. 9, 15285. doi: 10.1038/s41598-019-51601-2
Hina, F., Jin, Z., Yang, Z., Li, P., Fu, C. (2018). The complete chloroplast genome of Menispermum dauricum (Menispermaceae, Ranunculales). Mitochondrial DNA B Resour. 3, 913–914. doi: 10.1080/23802359.2018.1501306
Hina, F., Yisilam, G., Wang, S., Li, P., Fu, C. X. (2020). De novo transcriptome assembly, gene annotation and SSR marker development in the moon seed genus Menispermum (Menispermaceae). Front. Genet. 11. doi: 10.3389/fgene.2020.00380
Hong, Y. P., Chen, Z. D., Lu, A. M. (2001). Phylogeny of the tribe Menispermeae (Menispermaceae) reconstructed by ITS sequence data. Acta Phytotaxonomica Sin. 39, 97–104.
Hoot, S. B., Zautke, H., Harris, J. D., Crane, P. R., Neves, S. S. (2009). Phylogenetic patterns in Menispermaceae based on multiple chloroplast sequence data. Systematic Bot. 34, 44–56. doi: 10.1600/036364409787602339
Jacques, F. M. B., Gallut, C., Vignes-Lebbe, R., Zaragüeta I Bagils, R. (2008). Resolving phylogenetic reconstruction in Menispermaceae (Ranunculales) using fossils and a novel statistical test. Taxon 56, 379–392. doi: 10.1002/tax.562010
Jacques, F. M. B., Wang, W., Ortiz, R. D. C., Li, H. L., Zhou, Z. K., Chen, Z. D. (2011). Integrating fossils in a molecular-based phylogeny and testing them as calibration points for divergence time estimates in Menispermaceae. J. Systematics Evol. 49, 25–49. doi: 10.1111/j.1759-6831.2010.00105.x
Jiang, N., Zhou, Z., Yang, J. B., Yu, W. B. (2017). Complete chloroplast genome of Anemoclema glaucifolium (Ranunculaceae), a vulnerable and threatened species endemic to the hengduan mountains. Conserv. Genet. Resour. 10, 601–604. doi: 10.1007/s12686-017-0874-2
Jin, J. J., Yu, W. B., Yang, J. B., Song, Y., Depamphilis, C. W., Yi, T. S., et al. (2020). GetOrganelle: a fast and versatile toolkit for accurate de novo assembly of organelle genomes. Genome Biol. 21, 241. doi: 10.1186/s13059-020-02154-5
Kalyaanamoorthy, S., Minh, B. Q., Wong, T. K. F., von Haeseler, A., Jermiin, L. S. (2017). ModelFinder: fast model selection for accurate phylogenetic estimates. Nat. Methods 14, 587–589. doi: 10.1038/nmeth.4285
Kang, H., Wang, Y. (2019). The complete chloroplast genome sequence of Pericampylus glaucus. Mitochondrial DNA B Resour. 4, 3963–3964. doi: 10.1080/23802359.2019.1688718
Kessler, P. J. A. (1993). “Menispermaceae,” in The families and genera of vascular plants. II. flowering plants. dicotyledons. magnoliid, hamamelid and caryophyllid families. Eds. Kubitzki, K., Rohwer, J. G., Bittrich, V. (Berlin, Germany: Springer-Verlag), 402–418.
Kim, J., Lee, J., Um, S., Choi, S., Kim, H., Chun, H. S., et al. (2020). The complete chloroplast genome of Sinomenium acutum (Menispermaceae). Mitochondrial DNA B Resour. 5, 2992–2993. doi: 10.1080/23802359.2020.1797570
Kim, S., Soltis, D. E., Soltis, P. S., Zanis, M. J., Sun, Y. (2004). Phylogenetic relationships among early-diverging eudicots based on four genes: were the eudicots ancestrally woody? Mol. Phylogenet. Evol. 31, 16–30. doi: 10.1016/j.ympev.2003.07.017
Kong, H., Liu, W., Yao, G., Gong, W. (2017). A comparison of chloroplast genome sequences in Aconitum (Ranunculaceae): a traditional herbal medicinal genus. PeerJ 5, e4018. doi: 10.7717/peerj.4018
Krukoff, B. A., Moldenke, H. N. (1938). Studies of American Menispermaceae, with special reference to species used in preparation of arrow-poisons. Brittonia 3, 1–74. doi: 10.2307/2804992
Kundu, B. C., Guha, S. (1980). New species and variety of the genus Menispermum (Menispermaceae). Adansonia n.s. 20, 211–228.
Leigh, J. W., Bryant, D., Nakagawa, S. (2015). Popart: full-feature software for haplotype network construction. Methods Ecol. Evol. 6, 1110–1116. doi: 10.1111/2041-210x.12410
Li, X., Zhao, Y., Tu, X., Li, C., Zhu, Y., Zhong, H., et al. (2021). Comparative analysis of plastomes in Oxalidaceae: Phylogenetic relationships and potential molecular markers. Plant Diversity 43, 281–291. doi: 10.1016/j.pld.2021.04.004
Lian, L., Ortiz, D. C. R., Jabbour, F., Zhang, C. F., Xiang, X. G., Erst, A. S., et al. (2020). Phylogeny and biogeography of Pachygoneae (Menispermaceae), with consideration of the boreotropical flora hypothesis and resurrection of the genera Cebatha and Nephroia. Mol. Phylogenet. Evol. 148, 106825. doi: 10.1016/j.ympev.2020.106825
Liu, H. J., Ding, C. H., He, J., Cheng, J., Pei, L. Y., Xie, L. (2018a). Complete chloroplast genomes of Archiclematis, Naravelia and Clematis (Ranunculaceae), and their phylogenetic implications. Phytotaxa 343. doi: 10.11646/phytotaxa.343.3.2
Liu, H. J., He, J., Ding, C. H., Lyu, R. D., Pei, L. Y., Cheng, J., et al. (2018b). Comparative analysis of complete chloroplast genomes of Anemoclema, Anemone, Pulsatilla, and Hepatica revealing structural variations among genera in tribe Anemoneae (Ranunculaceae). Front. Plant Sci. 9. doi: 10.3389/fpls.2018.01097
Miers, J. (1851). A few remarks on the Menispermaceae. Ann. Magazine Natural History Ser. II 7, 33–45.
Moore, M. J., Soltis, P. S., Bell, C. D., Burleigh, J. G., Soltis, D. E. (2010). Phylogenetic analysis of 83 plastid genes further resolves the early diversification of eudicots. Proc. Natl. Acad. Sci. 107, 4623–4628. doi: 10.1073/pnas.0907801107
Morin, N. R. (1997). “Flora of north America,” in Magnoliophyta: Magnoliidae and hamamelidae, vol. 3. (Oxford, United Kingdom: Oxford University Press).
Ortiz, R. D. C., Kellogg, E. A., Werff, H. V. D. (2007). Molecular phylogeny of the moonseed family (Menispermaceae): implications for morphological diversification. Am. J. Bot. 94, 1425–1438. doi: 10.3732/ajb.94.8.1425
Ortiz, R. D. C., Wang, W., Jacques, F. M. B., Chen, Z. (2016). Phylogeny and a revised tribal classification of Menispermaceae (moonseed family) based on molecular and morphological data. Taxon 65, 1288–1312. doi: 10.12705/656.5
Prantl, K. (1888). “Menispermaceae,” in Dienatürlichen pflanzenfamilien, teil 3, abteilung 2. Eds. Engler, A., Prantl, K. (Germany, Leipzig: Engelman press), 78–91.
Purrington, F. F., Horn, D. J. (1993). Canada Moonseed vine (Menispermaceae): Host of four roundheaded wood borers in central Ohio (Coleoptera: Cerambycidae). Proc. Entomol. Soc. Washington 95, 313–320.
Ronquist, F., Teslenko, M., van der Mark, P., Ayres, D. L., Darling, A., Hohna, S., et al. (2012). MrBayes 3.2: Efficient Bayesian phylogenetic inference and model choice across a large model space. Systematic Biol. 61, 539–542. doi: 10.1093/sysbio/sys029
Rose, J. N. (1911). Studies of Mexican and central American plants. Contributions United States Natl. Herbarium 13, 302.
Rozas, J., Ferrer-Mata, A., Sanchez-Delbarrio, J. C., Guirao-Rico, S., Librado, P., Ramos-Onsins, S. E., et al. (2017). DnaSP 6: DNA sequence polymorphism analysis of Large data sets. Mol. Biol. Evol. 34, 3299–3302. doi: 10.1093/molbev/msx248
Shi, L., Chen, H., Jiang, M., Wang, L., Wu, X., Huang, L., et al. (2019). CPGAVAS2, an integrated plastome sequence annotator and analyzer. Nucleic Acids Res. 47, W65–W73. doi: 10.1093/nar/gkz345
Singh, B., Bedi, Y. S. (2016). Rediscovery, taxonomic history and extended enumeration of Haematocarpus validus bakh.f. ex forman (Menispermaceae) to indo-Myanmar biodiversity hotspot. Natl. Acad. Sci. Lett. 39, 383–387. doi: 10.1007/s40009-016-0483-8
Song, S., Zubov, D., Comes, H. P., Li, H., Liu, X., Zhong, X., et al. (2022). Plastid phylogenomics and plastome evolution of Nandinoideae (Berberidaceae). Front. Plant Sci. 13. doi: 10.3389/fpls.2022.913011
Stamatakis, A. (2014). RAxML version 8: a tool for phylogenetic analysis and post-analysis of large phylogenies. Bioinformatics 30, 1312–1313. doi: 10.1093/bioinformatics/btu033
Sun, Y., Moore, M. J., Landis, J. B., Lin, N., Chen, L., Deng, T., et al. (2018). Plastome phylogenomics of the early-diverging eudicot family Berberidaceae. Mol. Phylogenet. Evol. 128, 203–211. doi: 10.1016/j.ympev.2018.07.021
Sun, Y., Moore, M. J., Zhang, S., Soltis, P. S., Soltis, D. E., Zhao, T., et al. (2016). Phylogenomic and structural analyses of 18 complete plastomes across nearly all families of early-diverging eudicots, including an angiosperm-wide analysis of IR gene content evolution. Mol. Phylogenet. Evol. 96, 93–101. doi: 10.1016/j.ympev.2015.12.006
Wang, W., Chen, Z.-D., Liu, Y., Li, R.-Q., Li, J.-H. (2007a). Phylogenetic and biogeographic diversification of Berberidaceae in the northern hemisphere. Systematic Bot. 32, 731–742. doi: 10.1600/036364407783390791
Wang, W., Wang, H. C., Chen, Z. D. (2007b). Phylogeny and morphological evolution of tribe Menispermeae (Menispermaceae) inferred from chloroplast and nuclear sequences. Perspect. Plant Ecol. Evol. Systematics 8, 141–154. doi: 10.1016/j.ppees.2006.12.001
Wang, H. X., Liu, H., Moore, M. J., Landrein, S., Liu, B., Zhu, Z. X., et al. (2020). Plastid phylogenomic insights into the evolution of the Caprifoliaceae s.l. (Dipsacales). Mol. Phylogenet. Evol. 142, 106641. doi: 10.1016/j.ympev.2019.106641
Wang, W., Ortiz, R. D. C., Jacques, F. M. B., Xiang, X. G., Li, H. L., Lin, L., et al. (2012). Menispermaceae and the diversification of tropical rainforests near the Cretaceous–paleogene boundary. New Phytol. 195, 470–478. doi: 10.1111/j.1469-8137.2012.04158.x
Wefferling, K. M., Hoot, S. B., Neves, S. S. (2013). Phylogeny and fruit evolution in Menispermaceae. Am. J. Bot. 100, 883–905. doi: 10.3732/ajb.1200556
Wen, G., Chen, S., Fan, L., Zeng, L., Xu, B. (2021). The complete chloroplast genome of Arcangelisia gusanlung H.S.Lo (Menispermaceae), an important traditional medicine from south China. Mitochondrial DNA B Resour. 6, 3269–3270. doi: 10.1080/23802359.2021.1993099
Wick, R. R., Schultz, M. B., Zobel, J., Holt, K. E. (2015). Bandage: interactive visualization of de novo genome assemblies. Bioinformatics 31, 3350–3352. doi: 10.1093/bioinformatics/btv383
Wu, Z., Raven, P. H., Hong, D. (2008). “Flora of China,” in Menispermaceae through Capparaceae (Beijing: Science Press of China).
Xiang, K. L., Erst, A. S., Yang, J., Peng, H. W., Ortiz, R. D. C., Jabbour, F., et al. (2021). Biogeographic diversification of Eranthis (Ranunculaceae) reflects the geological history of the three great Asian plateaus. Proc. R. Soc. B: Biol. Sci. 288, 20210281. doi: 10.1098/rspb.2021.0281
Xiang, Q. Y., Soltis, D. E., Soltis, P. S., Manchester, S. R., Crawford, D. J. (2000). Timing the eastern Asian-eastern north American floristic disjunction: molecular clock corroborates paleontological estimates. Mol. Phylogenet. Evol. 15, 462–472. doi: 10.1006/mpev.2000.0766
Zhai, W., Duan, X., Zhang, R., Guo, C., Li, L., Xu, G., et al. (2019). Chloroplast genomic data provide new and robust insights into the phylogeny and evolution of the Ranunculaceae. Mol. Phylogenet. Evol. 135, 12–21. doi: 10.1016/j.ympev.2019.02.024
Zhang, Y. M., Han, L. J., Yang, C. W., Yin, Z. L., Tian, X., Qian, Z. G., et al (2021). Comparative chloroplast genome analysis of medicinally important Veratrum (Melanthiaceae) in China: Insights into genomic characterization and phylogenetic relationships. Plant Divers 43, 420–425. doi: 10.1016/j.pld.2021.05.004
Zhang, M. H., Wang, C. Y., Zhang, C., Zhang, D. C., Li, K. G., Nie, Z. L., et al. (2019). Phylogenetic relationships and biogeographic history of the unique Saxifraga sect. Irregulares (Saxifragaceae) from eastern Asia. J. Systematics Evol. 00 (0), 1–14. doi: 10.1111/jse.12547
Keywords: disjunct distribution, Menispermaceae, plastome evolution, phylogeny, systematic
Citation: Song S, Cameron KM, Wang Y, Wang S, Jin X, Hina F, Yang Z and Li P (2023) Phylogenomics and phylogeography of Menispermum (Menispermaceae). Front. Plant Sci. 14:1116300. doi: 10.3389/fpls.2023.1116300
Received: 05 December 2022; Accepted: 31 January 2023;
Published: 22 February 2023.
Edited by:
Nikolai Borisjuk, Huaiyin Normal University, ChinaReviewed by:
Xiaohua Jin, Institute of Botany (CAS), ChinaAnton Stepanenko, National Academy of Sciences of Ukraine, Ukraine
Copyright © 2023 Song, Cameron, Wang, Wang, Jin, Hina, Yang and Li. This is an open-access article distributed under the terms of the Creative Commons Attribution License (CC BY). The use, distribution or reproduction in other forums is permitted, provided the original author(s) and the copyright owner(s) are credited and that the original publication in this journal is cited, in accordance with accepted academic practice. No use, distribution or reproduction is permitted which does not comply with these terms.
*Correspondence: Zhaoping Yang, yzpzky@163.com; Pan Li, panli_zju@126.com